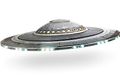

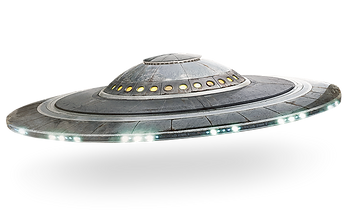
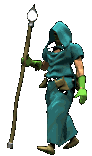
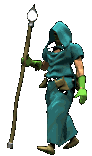
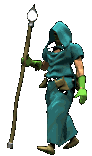
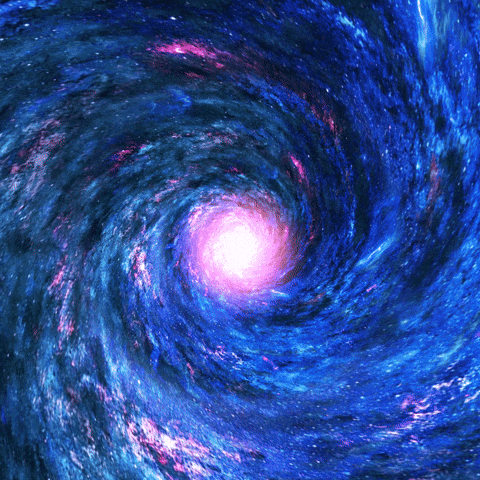
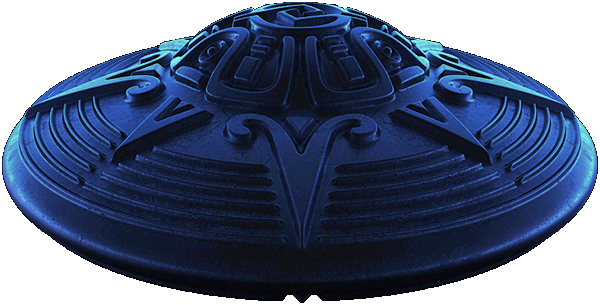
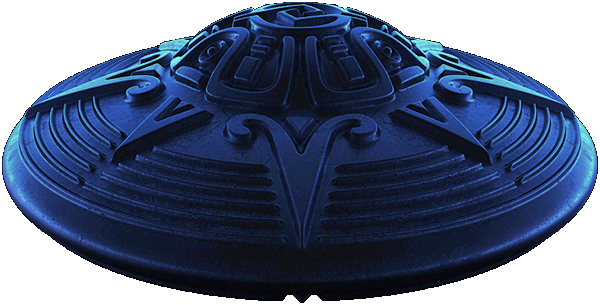
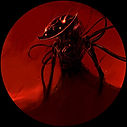
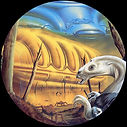

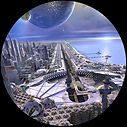
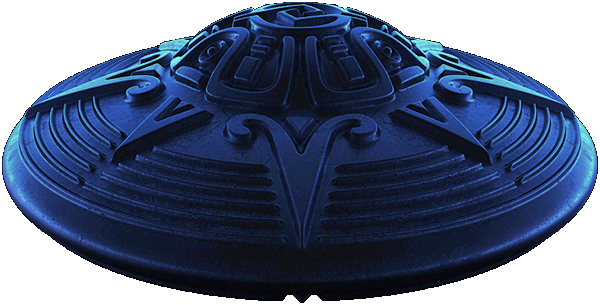
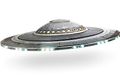
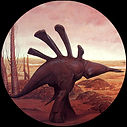
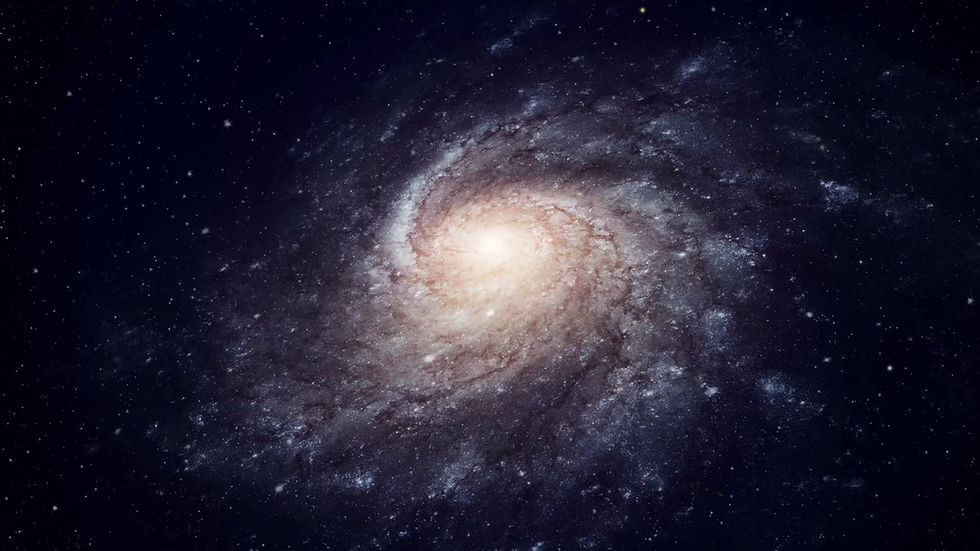
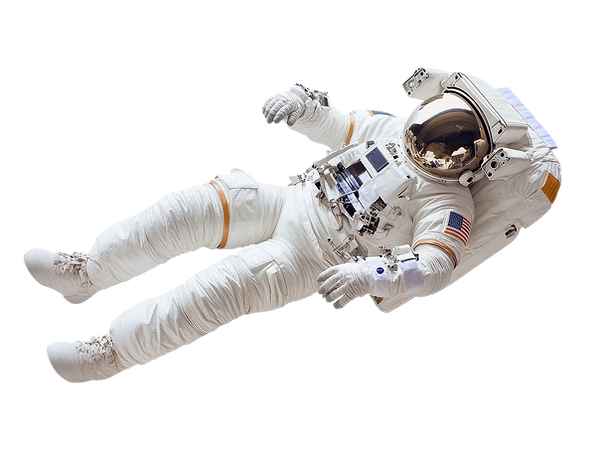
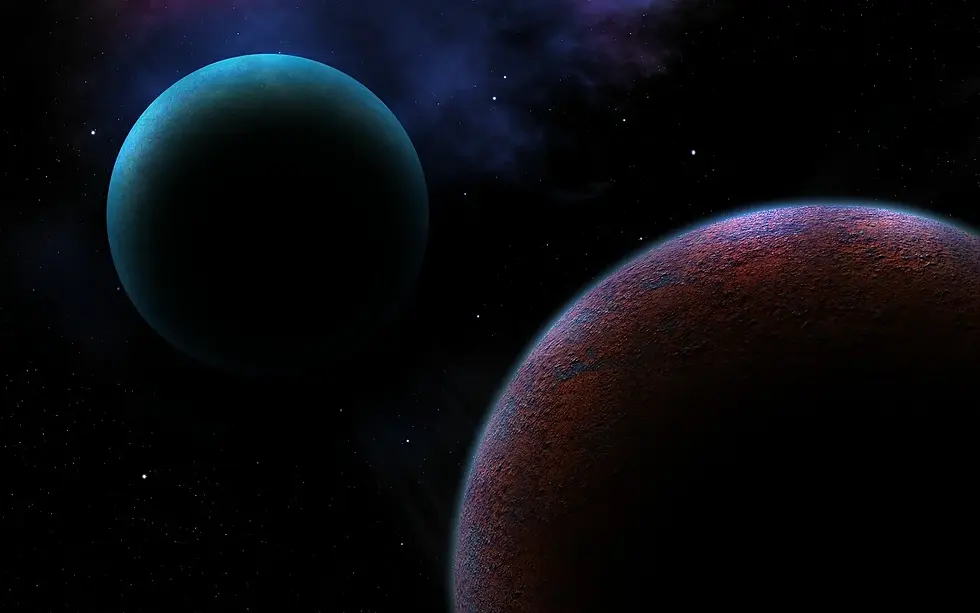
THE COSMAS
Galaxies consist of stars, planets, and vast clouds of gas and dust, all bound together by gravity. The largest contain trillions of stars and can be more than a million light-years across. The smallest can contain a few thousand stars and span just a few hundred light-years. Most large galaxies have supermassive black holes at their centers, some with billions of times the Sun’s mass.
Galaxies come in a variety of shapes, mostly spirals and ellipticals, as well as those with less orderly appearances, usually dubbed irregular.
Most galaxies are between 10 billion and 13.6 billion years old. Some are almost as old as the universe itself, which formed around 13.8 billion years ago. Astronomers think the youngest known galaxy formed approximately 500 million years ago.
Galaxies can organize into groups of about 100 or fewer members held together by their mutual gravity. Larger structures, called clusters, may contain thousands of galaxies. Groups and clusters can be arranged in superclusters, which are not gravitationally bound. Superclusters, empty voids, “walls” of galaxies, and other large-scale structures make up the cosmic web of matter in the universe.
Our Milky Way
Our home galaxy is called the Milky Way. It’s a spiral galaxy with a disk of stars spanning more than 100,000 light-years. Earth is located along one of the galaxy’s spiral arms, about halfway from the center. Our solar system takes about 240 million years to orbit the Milky Way just once.
This illustration shows the Milky Way, our home galaxy.
NASA/JPL-Caltech
From our perspective on Earth, the Milky Way looks like a faint, milky band of light arcing across the entire sky, which is how it got its name. This feature marks the central disk of our home galaxy seen edge on.
The Milky Way sits in a neighborhood with over 50 other galaxies called the Local Group. Its members range in size from dwarf galaxies (smaller galaxies with up to a few billion stars) to Andromeda, our nearest large galactic neighbor.
The Local Group sits just off the edge of the Virgo cluster and is part of the Laniakea supercluster.
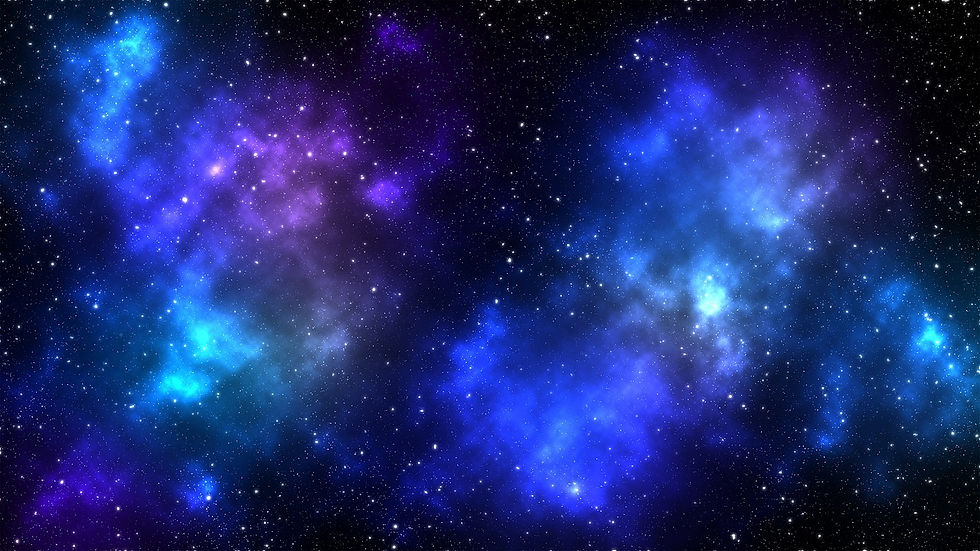
Black holes are among the most mysterious cosmic objects, much studied but not fully understood. These objects aren’t really holes. They’re huge concentrations of matter packed into very tiny spaces. A black hole is so dense that gravity just beneath its surface, the event horizon, is strong enough that nothing – not even light – can escape. The event horizon isn’t a surface like Earth’s or even the Sun’s. It’s a boundary that contains all the matter that makes up the black hole.
There is much we don’t know about black holes, like what matter looks like inside their event horizons. However, there is a lot that scientists do know about black holes.
Unable to render the provided source
Finding Black Holes
Black holes don’t emit or reflect light, making them effectively invisible to telescopes. Scientists primarily detect and study them based on how they affect their surroundings:
-
Black holes can be surrounded by rings of gas and dust, called accretion disks, that emit light across many wavelengths, including X-rays.
-
A supermassive black hole’s intense gravity can cause stars to orbit around it in a particular way. Astronomers tracked the orbits of several stars near the center of the Milky Way to prove it houses a supermassive black hole, a discovery that won the 2020 Nobel Prize.
-
When very massive objects accelerate through space, they create ripples in the fabric of space-time called gravitational waves. Scientists can detect some of these by the ripples’ effect on detectors.
-
Massive objects like black holes can bend and distort light from more distant objects. This effect, called gravitational lensing, can be used to find isolated black holes that are otherwise invisible.
Black Holes Are Not ...
-
Wormholes. They don’t provide shortcuts between different points in space, or portals to other dimensions or universes.
-
Cosmic vacuum cleaners. Black holes don’t suck in other matter. From far enough away, their gravitational effects are just like those of other objects of the same mass.
​​
An effort to find some of the biggest, most active black holes in the universe provides a better estimate for the ratio of hidden to unhidden behemoths.
Multiple NASA telescopes recently helped scientists search the sky for supermassive black holes — those up to billions of times heavier than the Sun. The new survey is unique because it was as likely to find massive black holes that are hidden behind thick clouds of gas and dust as those that are not.
Astronomers think that every large galaxy in the universe has a supermassive black hole at its center. But testing this hypothesis is difficult because researchers can’t hope to count the billions or even trillions of supermassive black holes thought to exist in the universe. Instead they have to extrapolate from smaller samples to learn about the larger population. So accurately measuring the ratio of hidden supermassive black holes in a given sample helps scientists better estimate the total number of supermassive black holes in the universe.
The new study published in the Astrophysical Journal found that about 35% of supermassive black holes are heavily obscured, meaning the surrounding clouds of gas and dust are so thick they block even low-energy X-ray light. Comparable searches have previously found less than 15% of supermassive black holes are so obscured. Scientists think the true split should be closer to 50/50 based on models of how galaxies grow. If observations continue to indicate significantly less than half of supermassive black holes are hidden, scientists will need to adjust some key ideas they have about these objects and the role they play in shaping galaxies.
Hidden Treasure
Although black holes are inherently dark — not even light can escape their gravity — they can also be some of the brightest objects in the universe: When gas gets pulled into orbit around a supermassive black hole, like water circling a drain, the extreme gravity creates such intense friction and heat that the gas reaches hundreds of thousands of degrees and radiates so brightly it can outshine all the stars in the surrounding galaxy.
The clouds of gas and dust that surround and replenish the bright central disk may roughly take the shape of a torus, or doughnut. If the doughnut hole is facing toward Earth, the bright central disk within it is visible; if the doughnut is seen edge-on, the disk is obscured.
A supermassive black hole surrounded by a torus of gas and dust is depicted in four different wavelengths of light in this artist’s concept. Visible light (top right) and low-energy X-rays (bottom left) are blocked by the torus; infrared (top left) is scattered and reemitted; and some high energy X-rays (bottom right) can penetrate the torus.
NASA/JPL-Caltech
Most telescopes can rather easily identify face-on supermassive black holes, though not edge-on ones. But there’s an exception to this that the authors of the new paper took advantage of: The torus absorbs light from the central source and reemits lower-energy light in the infrared range (wavelengths slightly longer than what human eyes can detect). Essentially, the doughnuts glow in infrared.
These wavelengths of light were detected by NASA’s Infrared Astronomical Satellite, or IRAS, which operated for 10 months in 1983 and was managed by NASA’s Jet Propulsion Laboratory in Southern California. A survey telescope that imaged the entire sky, IRAS was able to see the infrared emissions from the clouds surrounding supermassive black holes. Most importantly, it could spot edge-on and face-on black holes equally well.
IRAS caught hundreds of initial targets. Some of them turned out to be not heavily obscured black holes but galaxies with high rates of star formation that emit a similar infrared glow. So the authors of the new study used ground-based, visible-light telescopes to identify those galaxies and separate them from the hidden black holes.
To confirm edge-on, heavily obscured black holes, the researchers relied on NASA’s NuSTAR (Nuclear Spectroscopic Telescope Array), an X-ray observatory also managed by JPL. X-rays are radiated by some of the hottest material around the black hole. Lower-energy X-rays are absorbed by the surrounding clouds of gas and dust, while the higher-energy X-rays observed by NuSTAR can penetrate and scatter off the clouds. Detecting these X-rays can take hours of observation, so scientists working with NuSTAR first need a telescope like IRAS to tell them where to look.
NASA’s NuSTAR X-ray telescope, depicted in this artist’s concept, has helped astronomers get a better sense of how many supermassive black holes are hidden from view by thick clouds of gas and dust that surround them.
NASA/JPL-Caltech
“It amazes me how useful IRAS and NuSTAR were for this project, especially despite IRAS being operational over 40 years ago,” said study lead Peter Boorman, an astrophysicist at Caltech in Pasadena, California. “I think it shows the legacy value of telescope archives and the benefit of using multiple instruments and wavelengths of light together.”
Numerical Advantage
Determining the number of hidden black holes compared to nonhidden ones can help scientists understand how these black holes get so big. If they grow by consuming material, then a significant number of black holes should be surrounded by thick clouds and potentially obscured. Boorman and his coauthors say their study supports this hypothesis.
In addition, black holes influence the galaxies they live in, mostly by impacting how galaxies grow. This happens because black holes surrounded by massive clouds of gas and dust can consume vast — but not infinite — amounts of material. If too much falls toward a black hole at once, the black hole starts coughing up the excess and firing it back out into the galaxy. That can disperse gas clouds within the galaxy where stars are forming, slowing the rate of star formation there.
“If we didn’t have black holes, galaxies would be much larger,” said Poshak Gandhi, a professor of astrophysics at the University of Southampton in the United Kingdom and a coauthor on the new study. “So if we didn’t have a supermassive black hole in our Milky Way galaxy, there might be many more stars in the sky. That’s just one example of how black holes can influence a galaxy’s evolution.”
More About NuSTAR
A Small Explorer mission led by Caltech and managed by NASA’s Jet Propulsion Laboratory in Southern California for the agency’s Science Mission Directorate in Washington, NuSTAR was developed in partnership with the Danish Technical University and the Italian Space Agency (ASI). The spacecraft was built by Orbital Sciences Corp. in Dulles, Virginia. NuSTAR’s mission operations center is at the University of California, Berkeley, and the official data archive is at NASA’s High Energy Astrophysics Science Archive Research Center at NASA’s Goddard Space Flight Center.
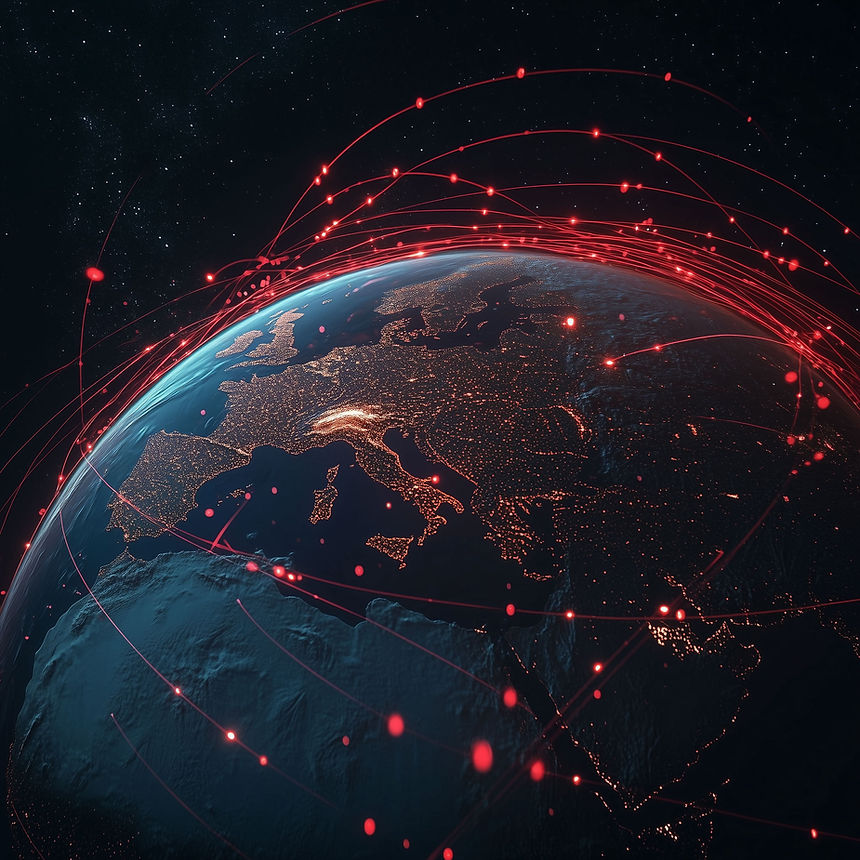
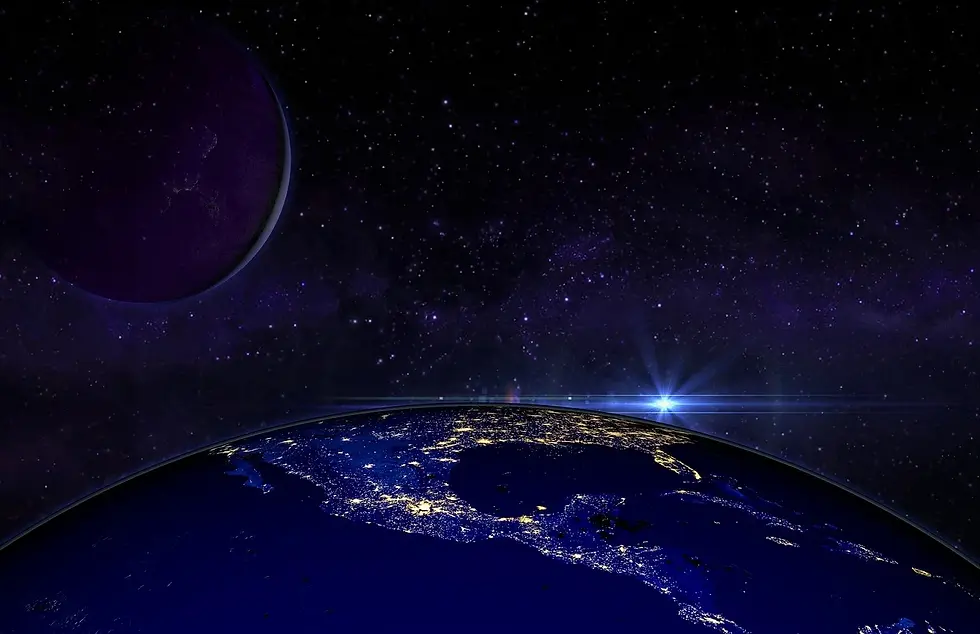
Super-Earth Basics
Super-Earths – a class of planets unlike any in our solar system – are more massive than Earth yet lighter than ice giants like Neptune and Uranus, and can be made of gas, rock or a combination of both. They are between twice the size of Earth and up to 10 times its mass.
Super-Earth is a reference only to an exoplanet’s size – larger than Earth and smaller than Neptune – but not suggesting they are necessarily similar to our home planet. The true nature of these planets remains shrouded in uncertainty because we have nothing like them in our own solar system – and yet, they are common among planets found so far in our galaxy.
Over the last three decades, we have discovered all kinds of strange planets we never knew existed and that have no analog in our solar system. Super-Earths can be up to 10 times more massive than Earth. We don’t yet know enough about these planets to tell at what point they might lose a rocky surface. But in the range of 3-10 times the mass of Earth, there might be a wide variety of planetary compositions, including water worlds, snowball planets, or planets that, like Neptune, are composed largely of dense gas. Exoplanets at the upper limits of the super-Earth size limit can also be referred to as sub-Neptunes, or mini-Neptunes.
Neptunian exoplanets are similar in size to Neptune or Uranus in our solar system. Neptunian planets typically have hydrogen and helium-dominated atmospheres with cores of rock and heavier metals.
Neptunian exoplanets are similar in size to Neptune or Uranus in our solar system. (Neptune is about four times the size, or radius, of Earth and almost 17 times its mass, or weight.) Neptunian exoplanets may have a mixture of interiors though all would be rocky with heavier metals at their cores. Neptunian planets typically have hydrogen- and helium-dominated atmospheres. We’re also discovering mini-Neptunes, planets smaller than Neptune and bigger than Earth. No planets like these exist in our solar system.
While Uranus and Neptune are mostly composed of hydrogen and helium, both also contain water, ammonia, and methane. Since these three chemicals are typically found frozen as ices in the cold outer solar system, Uranus and Neptune are often referred to as "ice giants" (though their interiors are warm enough that the "ices" inside them are not frozen). Researchers discovered an ice giant exoplanet 25,000 light-years away in 2014. We don’t know much about its composition, what it’s made of, or what elements are present in its atmosphere, but it’s in a similar orbit to its star as Uranus is to our Sun.
It can be hard to tell a lot about distant planets’ compositions. Space telescopes like NASA’s Hubble (and formerly Spitzer) glean information about planet atmospheres by analyzing starlight. As starlight passes through a planet's atmosphere, atoms and molecules absorb light at certain wavelengths, blocking it from the telescope's view. The more light a planet blocks, the larger the planet appears. By analyzing the amount of light blocked by the planet at different wavelengths, called spectroscopy, researchers can determine which molecules make up the atmosphere. NASA’s Transiting Exoplanet Survey Satellite (TESS) is designed to discover planets smaller than Neptune that transit stars bright enough to enable follow-up spectroscopic observations that can provide atmospheric compositions.
Neptunian exoplanets often have thick clouds that block any light from coming through, hiding the signature of the molecules in the atmosphere. The James Webb Space Telescope, launched in 2021, is getting our best looks yet at exoplanet atmospheres.
Scientists found clear skies on an exoplanet about the size of Neptune, using the combined power of NASA's Hubble, Spitzer, and Kepler space telescopes. The view from this planet – were it possible to fly a spaceship into its gaseous layers – is illustrated at right. The illustrated view at left shows the high cloud layers of many Neptunian exoplanets that block the ability to detect molecules in the planets’ atmospheres.
NASA/JPL-Caltech
Astronomers were elated to find clear skies on a Neptune-size planet called HAT-P-11b in 2017. Without clouds to block the view, they were able to identify water vapor molecules in the exoplanet's atmosphere. HAT-P-11b is gaseous with a rocky core, much like our own Neptune. Its atmosphere may have clouds deeper down, but the combined observations from Hubble, Spitzer, and Kepler showed that the upper region is cloud-free. This good visibility enabled scientists to detect water vapor molecules in the planet's atmosphere.
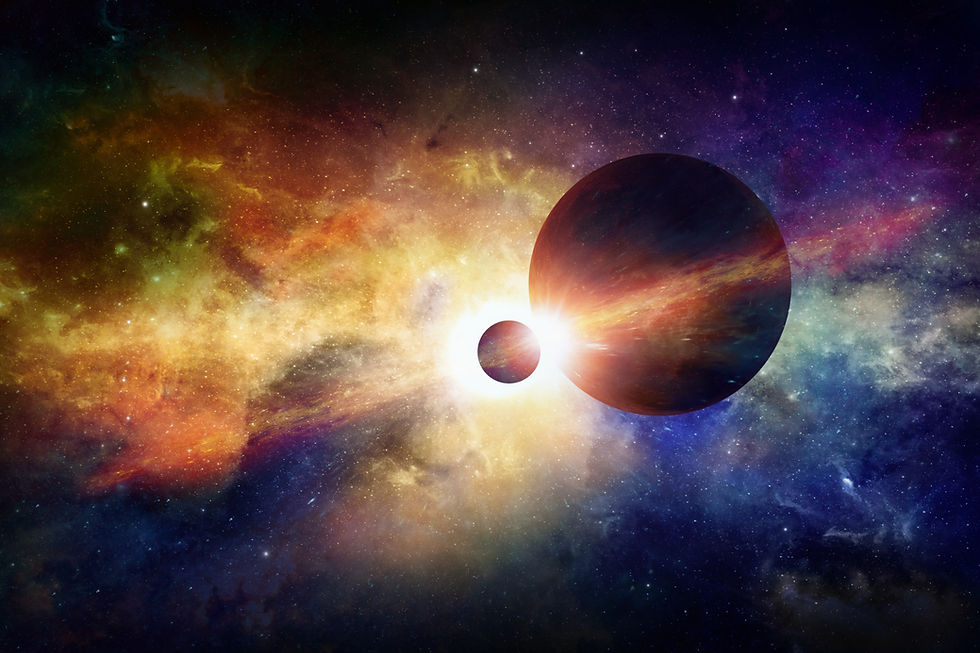
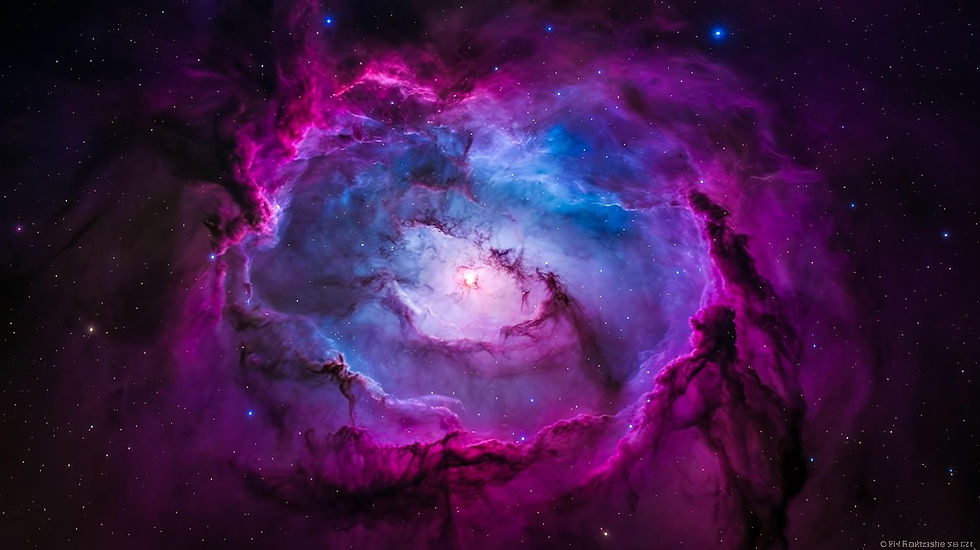
Astronomers estimate that the universe could contain up to one septillion stars – that’s a one followed by 24 zeros. Our Milky Way alone contains more than 100 billion, including our most well-studied star, the Sun.
Stars are giant balls of hot gas – mostly hydrogen, with some helium and small amounts of other elements. Every star has its own life cycle, ranging from a few million to trillions of years, and its properties change as it ages.
Birth
Stars form in large clouds of gas and dust called molecular clouds. Molecular clouds range from 1,000 to 10 million times the mass of the Sun and can span as much as hundreds of light-years. Molecular clouds are cold which causes gas to clump, creating high-density pockets. Some of these clumps can collide with each other or collect more matter, strengthening their gravitational force as their mass grows. Eventually, gravity causes some of these clumps to collapse. When this happens, friction causes the material to heat up, which eventually leads to the development of a protostar – a baby star. Batches of stars that have recently formed from molecular clouds are often called stellar clusters, and molecular clouds full of stellar clusters are called stellar nurseries.
The edge of a nearby stellar nursery called NGC 3324, found at the northwest corner of the Carina Nebula, forms the “mountains” and “valleys” spanning this image captured by the James Webb Space Telescope.
NASA, ESA, CSA, and STScI
Life
At first, most of the protostar’s energy comes from heat released by its initial collapse. After millions of years, immense pressures and temperatures in the star’s core squeeze the nuclei of hydrogen atoms together to form helium, a process called nuclear fusion. Nuclear fusion releases energy, which heats the star and prevents it from further collapsing under the force of gravity.
Our Sun, a main sequence star, emits a strong solar flare flashes in this image captured by NASA's Solar Dynamics Observatory.
NASA/SDO
Astronomers call stars that are stably undergoing nuclear fusion of hydrogen into helium main sequence stars. This is the longest phase of a star’s life. The star’s luminosity, size, and temperature will slowly change over millions or billions of years during this phase. Our Sun is roughly midway through its main sequence stage.
A star’s gas provides its fuel, and its mass determines how rapidly it runs through its supply, with lower-mass stars burning longer, dimmer, and cooler than very massive stars. More massive stars must burn fuel at a higher rate to generate the energy that keeps them from collapsing under their own weight. Some low-mass stars will shine for trillions of years – longer than the universe has currently existed – while some massive stars will live for only a few million years.
Death
At the beginning of the end of a star’s life, its core runs out of hydrogen to convert into helium. The energy produced by fusion creates pressure inside the star that balances gravity’s tendency to pull matter together, so the core starts to collapse. But squeezing the core also increases its temperature and pressure, making the star slowly puff up. However, the details of the late stages of the star’s death depend strongly on its mass.
A low-mass star’s atmosphere will keep expanding until it becomes a subgiant or giant star while fusion converts helium into carbon in the core. (This will be the fate of our Sun, in several billion years.) Some giants become unstable and pulsate, periodically inflating and ejecting some of their atmospheres. Eventually, all the star’s outer layers blow away, creating an expanding cloud of dust and gas called a planetary nebula.
The Helix nebula, imaged here, lies 650 light-years away in the constellation Aquarius. Also known as NGC 7293, it is a typical example of planetary nebulae.
NASA/JPL-Caltech
All that’s left of the star is its core, now called a white dwarf, a roughly Earth-sized stellar cinder that gradually cools over billions of years.
A high-mass star goes further. Fusion converts carbon into heavier elements like oxygen, neon, and magnesium, which will become future fuel for the core. For the largest stars, this chain continues until silicon fuses into iron. These processes produce energy that keeps the core from collapsing, but each new fuel buys it less and less time. The whole process takes just a few million years. By the time silicon fuses into iron, the star runs out of fuel in a matter of days. The next step would be fusing iron into some heavier element but doing so requires energy instead of releasing it.
The remnant of a supernova observed in 1572, notably studied by the Danish astronomer Tycho Brahe, lies about 13,000 light-years away in the constellation Cassiopeia. In this composite image, data from NASA’s Chandra X-ray Observatory have been combined with an optical image of stars in the same area.
X-ray: NASA/CXC/RIKEN & GSFC/T. Sato et al; Optical: DSS
The star’s iron core collapses until forces between the nuclei push the brakes, then it rebounds. This change creates a shock wave that travels outward through the star. The result is a huge explosion called a supernova. The core survives as an incredibly dense remnant, either a neutron star or a black hole.
Material cast into the cosmos by supernovae and other stellar events will enrich future molecular clouds and become incorporated into the next generation of stars.
