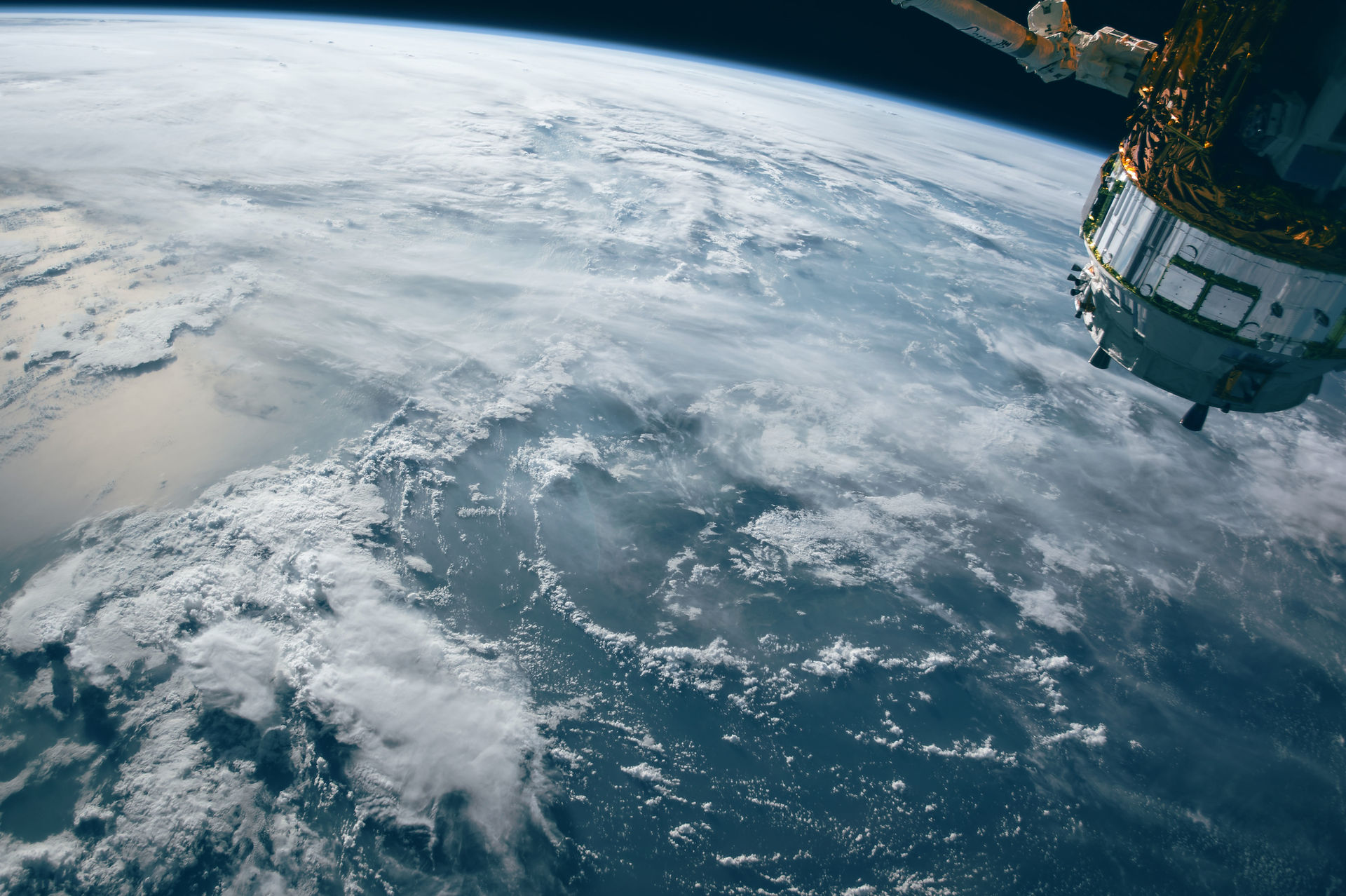
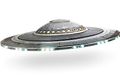

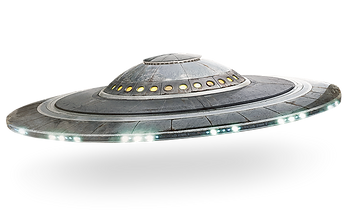
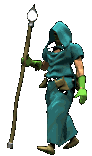
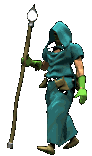
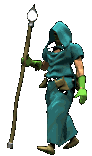
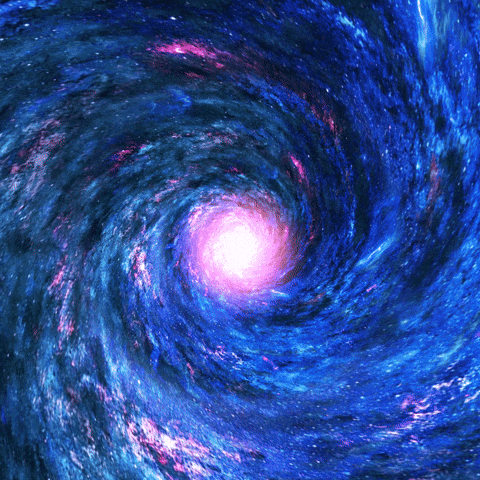
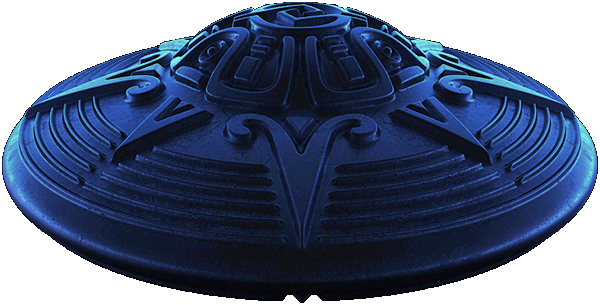
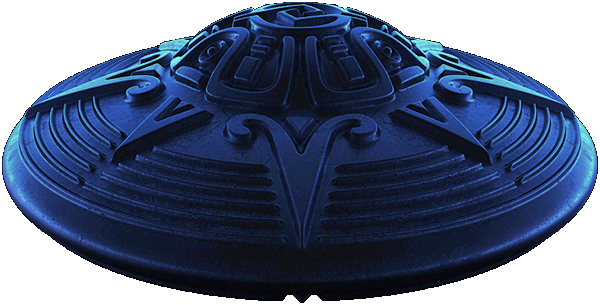
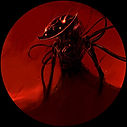
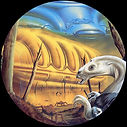

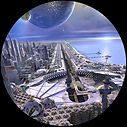
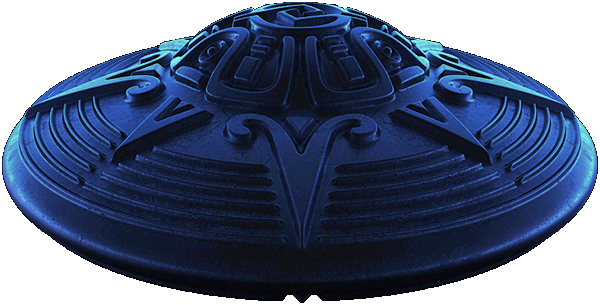
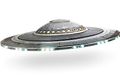
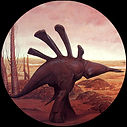
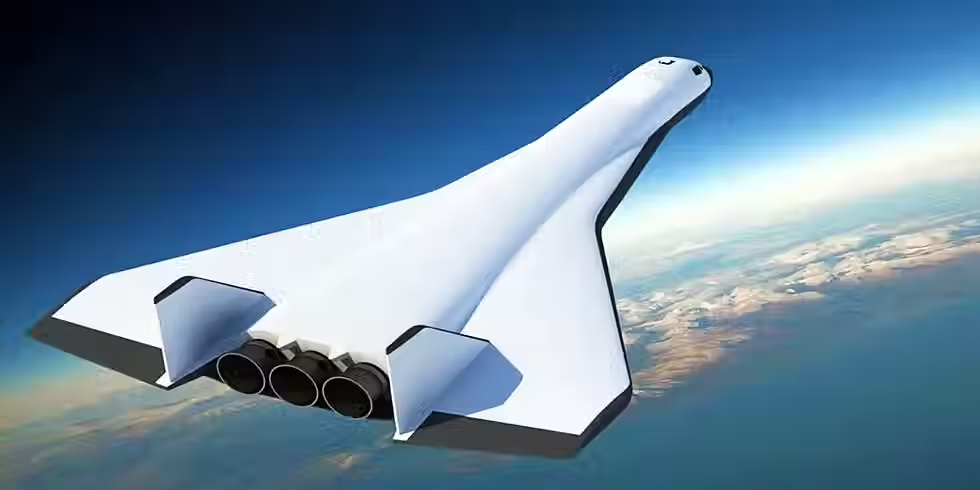
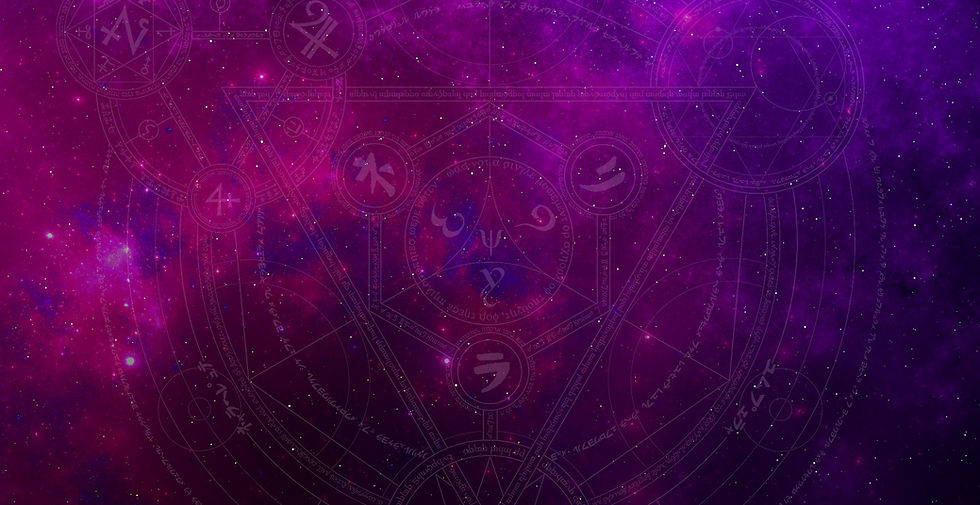
FUTURE SPACE SHIPS
The future of space exploration involves both telescopic and physical explorations of space by robotic spacecraft and human spaceflight. Near-term physical exploration missions, focused on obtaining new information about the Solar System, are planned and announced by both national and private organisations.
Tentative plans for crewed orbital and landing missions to the Moon and Mars to establish scientific outposts will later enable permanent and self-sufficient settlements. Further exploration will potentially involve expedition and the other planets and settlements on the Moon, as well as establishing mining and fueling outposts, particularly in the asteroid belt. Physical exploration outside the Solar System will be robotic for the foreseeable future.
Benefits of space exploration
Investment in space exploration has dramatically shifted since the 20th century Space race. Space exploration of the late 20th century was driven by competition between the Soviet Union and the United States to achieve the first spaceflight. Now, the private sector and national governments are again investing in space exploration. However, this time they are motivated by protecting human life from catastrophic events and leveraging the resources of space
Colonize outer space
It has been argued that space colonization is a means of ensuring the survival of human civilization given a planetary disaster. Colonizing other planets allows for the dispersal of humans and thus increases the likelihood of survival given a planetary disaster. The availability of additional resources that can be mined from space could potentially expand the capabilities of humans and largely benefit society. Leveraging these resources and moving high polluting industries to space could reduce the emissions on Earth and ultimately lead to finding cleaner energy sources. The primary blockers to colonizing space include technological and economic challenges.
Many private companies are working to make space travel more efficient in hopes to reduce the overall cost of space travel, and thus space colonization. SpaceX has been a dominant leader in this push for efficient exploration with the release of the Falcon 9, a reusable rocket.
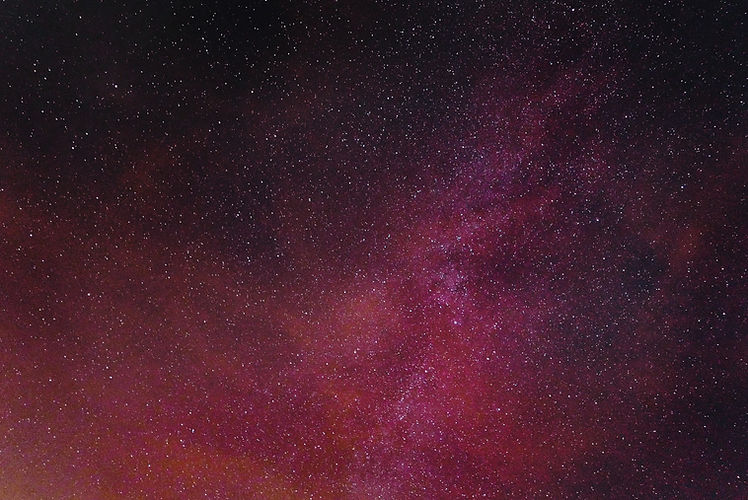
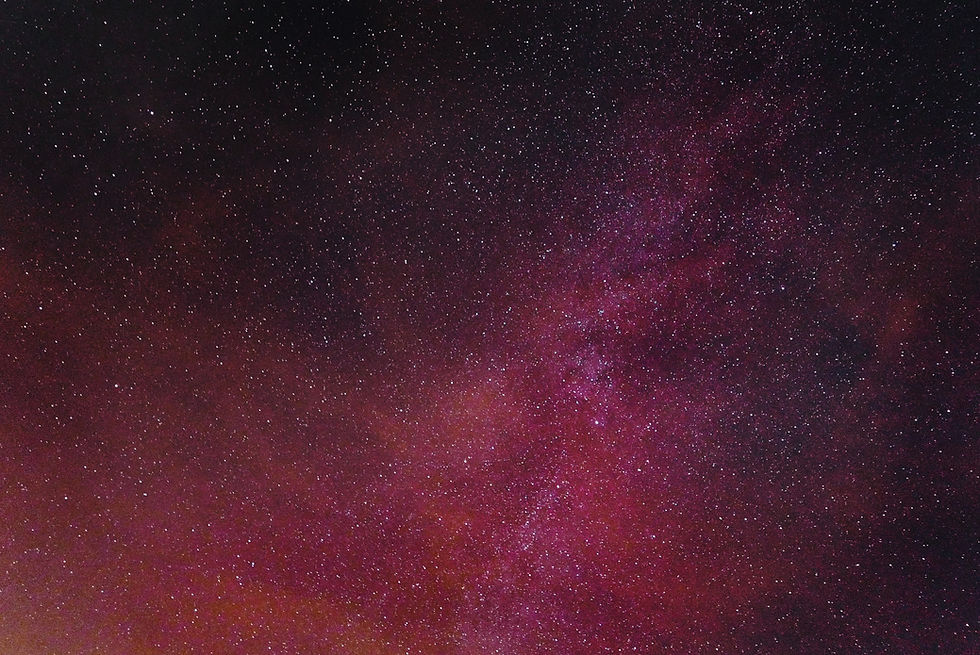
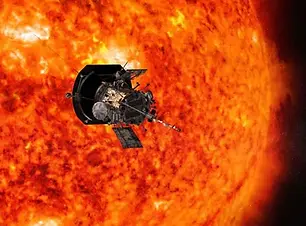
Space research
The unique attributes of space enable astronuts to conduct research that could not otherwise be done on Earth, and the perspective from space looking at Earth enables scientists to gain more insight on the Earth's natural environment. Research conducted at the International Space Station aims to benefit human civilizations on Earth and extend human knowledge of space and space exploration. Currently, NASA's research at the ISS includes biomedical research, material science, technology advancement, and methods to enable further space exploration.
Anti and microgravity enable astronauts to execute medical research that is impossible to perform on Earth. For example, NASA's research on new treatment options for complex diseases, such as Duchenne Muscular Dystrophy, require the use of a microgravity environment to allow the microparticles in the treatment solution to stay robust. NASA has also reported research investment in microbial vaccine development and microencapsulation of drugs for targeted and more efficient treatment delivery

Uncrewed missions
Breakthrough Starshot is a research and engineering project by the Breakthrough Initiatives to develop a proof-of-concept fleet of light sail spacecraft named StarChip, to be capable of making the journey to the Alpha Centauri star system 4.37 light-years away. It was founded in 2016 by Yuri Milner, Stephen Hawking, and Mark Zuckerberg.
Mars
Rosalind Franklin
Main article: Rosalind Franklin (rover)
Rosalind Franklin, previously known as the ExoMars rover, is a planned robotic Mars rover, part of the international ExoMars programme led by the European Space Agency and the Russian Roscosmos State Corporation.
Initially scheduled to launch in July 2020, but has since been delayed due to testing issues with the rover's landing mechanism. As of May 2022, the launch of the rover is not expected to occur before 2028 due to the need for a new non-Russian landing platform. Once safely landed, the solar powered rover will begin a seven-month (218-sol) mission to search for the existence of past life on Mars. The Trace Gas Orbiter (TGO), launched in 2016, will operate as Rosalind Franklin's and lander's data-relay satellite.
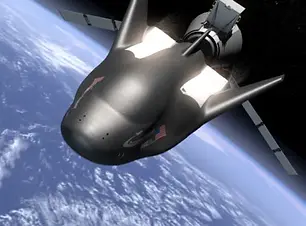
Mangalyaan 2
Mars Orbiter Mission 2 (MOM 2), also called Mangalyaan-2, is India's second interplanetary mission planned for launch to Mars by the Indian Space Research Organisation (ISRO). As per some reports emerged, the mission was to be an orbiter to Mars proposed for 2024. However, in a recorded interview in October 2019, VSSC director has indicated the inclusion of a lander and rover.[13] The orbiter will use aerobraking to lower its initial apoapsis and enter into an orbit more suitable for observations
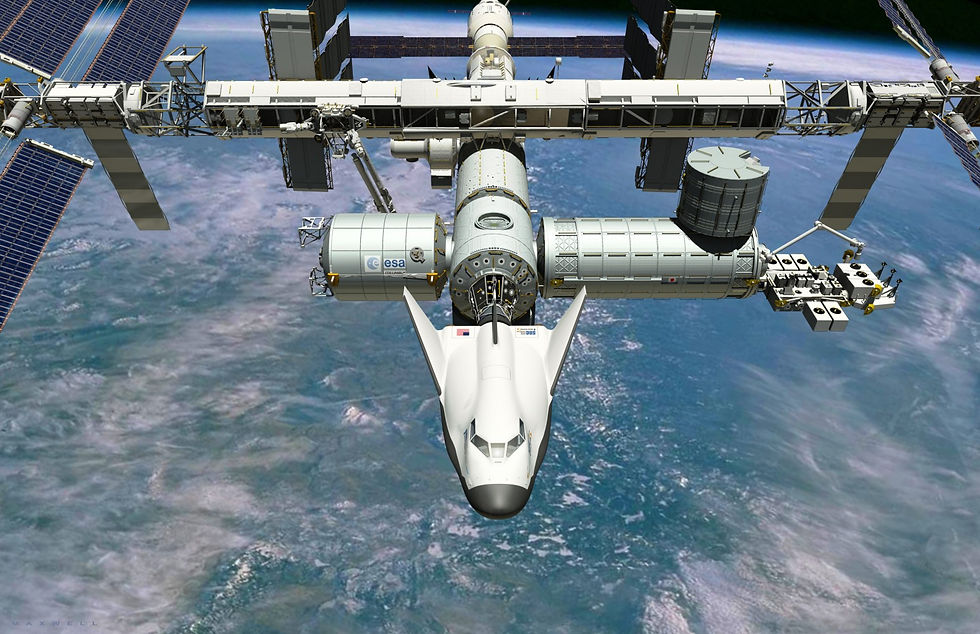

futuristic space technologies — and when they might happen
Space telescopes
PLATO
Planetary Transits and Oscillations of Stars (PLATO) is a space telescope under development by the European Space Agency for launch in 2026. The mission goals are to search for planetary transits across up to one million stars, and to discover and characterize rocky extrasolar planets around yellow dwarf stars (like the Sun), subgiant stars, and red dwarf stars. The emphasis of the mission is on Earth-like planets in the habitable zone around Sun-like stars where water can exist in liquid state. It is the third medium-class mission in ESA's Cosmic Vision programme and named after the influential Greek philosopher Plato, the founding figure of Western philosophy, science and mathematics. A secondary objective of the mission is to study stellar oscillations or seismic activity in stars to measure stellar masses and evolution and enabling the precise characterization of the planet host star, including its age
SpaceX Starship
The SpaceX Starship is planned to be a spacecraft launched as the second stage of a reusable launch vehicle. The concept is under development by SpaceX, as a private spaceflight project. It is being designed to be a long-duration cargo- and passenger-carrying spacecraft.While it will be tested on its own initially, it will be used on orbital launches with an additional booster stage, the Super Heavy, where Starship would serve as the second stage on a two-stage-to-orbit launch vehicle. The combination of spacecraft and booster is called Starship as well.
Boeing Starliner 1
The Boeing Starliner 1 mission will be the first operational crewed mission of the Boeing Starliner and the first mission to reuse the Starliner Spacecraft. The mission is expected to launch no earlier than December 2021 using the Atlas V rocket with a crew of four astronauts, three NASA astronauts and likely one international partner astronaut from either Japan, Canada, or the European Space Agency. This mission will be the fourth US spaceflight with a female commander.
Gaganyaan
ISRO's future Gaganyaan mission, which is the first Indian Human Spaceflight Programme, comprises a crew module which is a fully autonomous 5.3-tonne (12,000 lb) spacecraft designed to carry a 3-member crew to orbit and safely return to the Earth after a mission duration of up to seven days. Its 2.9-tonne (6,400 lb) service module is powered by liquid propellant engines. It is to be launched on the GSLV Mk III launcher no earlier than 2022. About 16 minutes after liftoff from the Satish Dhawan Space Centre (SDSC), Sriharikota, the rocket will inject the spacecraft into an orbit 300–400 km (190–250 mi) above Earth. When ready to land, its service module and solar panels will be disposed off before reentry. The capsule would return for a parachute splashdown in the Bay of Bengal.
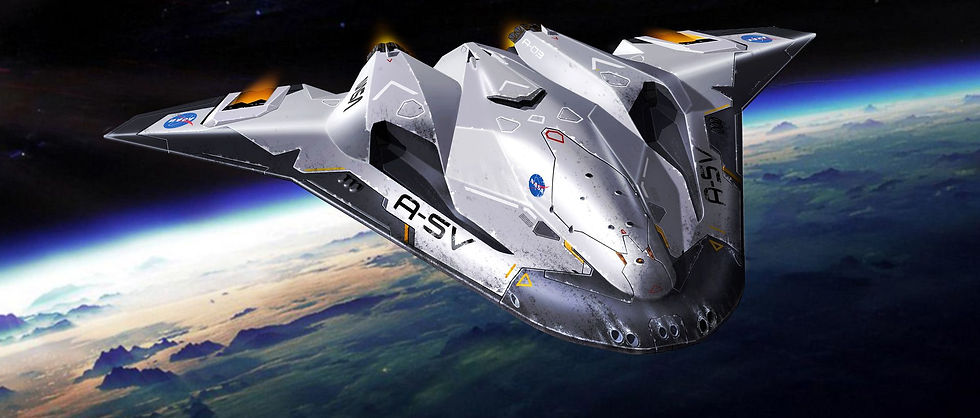
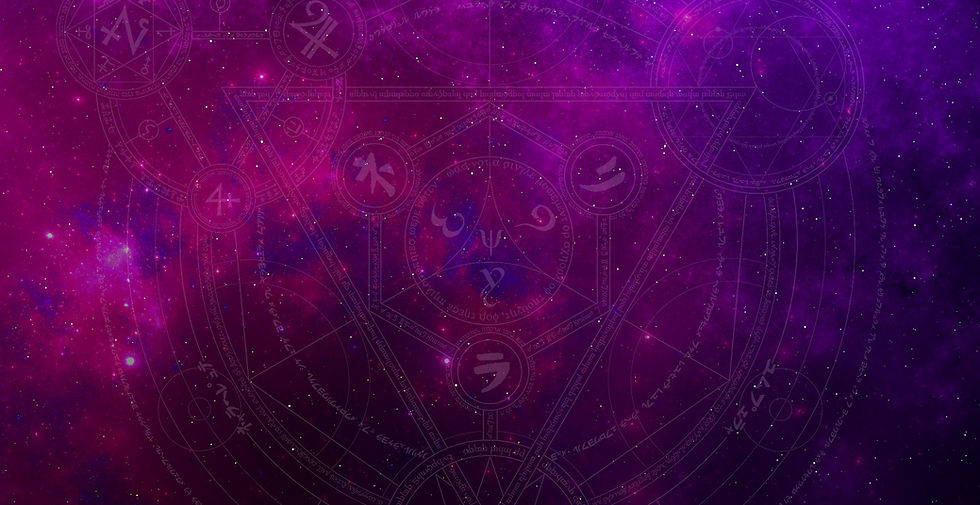
World Space Week this year, which runs between Oct. 4 and Oct. 10,is celebrating how space technology is aiding us in our fight against climate change on Earth — but sometimes it pays to also look outward at what technology can offer us as we expand into space to harness the energy and the worlds that lie out there.
Here we highlight four technologies, look at the challenges that they pose and give some indication of when they might come to fruition — if ever. The time estimates are not necessarily a prediction of when they might happen, but are intended to give a rough idea of how much work still needs to be done on them.
Related: Space-based solar power may be one step closer to reality, thanks to this key test
Currently, solar power provides just over 5% of the world's total electricity supply, but we can do much better than that.
You may like
-
Beam me to the stars: Scientists propose wild new interstellar travel tech
-
Trump wants the US to land astronauts on Mars soon. Could it happen by 2029?
The best place to feel the sun’s energy is in space, without clouds to block the view or an atmosphere to absorb our star's rays. A huge array of solar panels would therefore have an unfettered view of the sun, but the tricky part of this idea concerns building such a space-based array in the first place. Plus, even if we manage that somehow, how would we get the harvested solar energy down to Earth?
Compared to most technologies on this list, power beaming from space is actually ahead of the curve. In January of 2023, the Caltech-built Space Solar Power Demonstrator launched into Earth orbit. On board was an instrument called MAPLE, the Microwave Array for Power-transfer Low-orbit Experiment. MAPLE successfully converted solar energy into microwaves and then beamed the microwaves down to a receiving station at Caltech, where it was converted into electricity. It was a pretty low amount of power — just milliwatts — but it was an exciting proof of concept.Now, the Japanese Aerospace Agency, JAXA, working with commercial interests, are exploring their own program that the agency hopes to culminate in a solar farm capable of producing one gigawatt of energy and beaming it down to Earth. However, building a solar farm isn't easy.
A solar panel in space, above the absorbing effects of the atmosphere, receives about a kilowatt of energy per square meter (10 square feet) of the sun. Solar panels are not 100% efficient, however; currently available commercial models have an efficiency of just 30%, meaning a solar panel in space can realistically produce just 300 watts per square meter. To scale this up to producing 1 gigawatt of energy, which would be the equivalent of a nuclear power station on Earth, would require an enormous array of solar panels, multiple kilometers in diameter, with a mass of 10,000 metric tons. Compare this to the International Space Station, which has a mass of 419 metric tons, and that shows just what a daunting engineering task this would be.
Supposing a solar farm could be built in space, it would be placed in geosynchronous orbit, 35,786 km (22,236 miles) above the Earth. The challenge would then be to keep the microwave beam narrow and on target — you wouldn't want the microwave beam to stray and fry something accidentally. Although lasers instead of microwaves would be easier to direct, laser energy can be absorbed by water vapor in the atmosphere or blocked by clouds, whereas microwaves pass freely through them.
Lasers, though, might be more suitable for space-to-space power transfer. This could extend the life of satellites, for example, but they would have to be built with some kind of receiver to accept the incoming laser power beam. We could also imagine a network of solar farms and relay satellites around the moon, beaming power via lasers to a lunar base on the surface.
Second half of the 21st century: Space elevators
This is an old science fiction concept, first conceived by Russian scientist Konstantin Tsiolkovsky — rather than blasting off in a rocket atop a dangerous column of flame, why not ride into space on an elevator car?
The basic design of a space elevator sounds simple. A thick cable extends from a location within 10 degrees of the Earth's equator up into space. The forces acting on the cable would be fierce, with Earth's gravity trying to pull it down, and the centrifugal force on a mass at the end of the cable in Earth orbit pulling it the other way, keeping it taut. The stresses and tension of this cable would be so great that it would need to be made from a material 50 times stronger than steel, however. The only material strong enough are carbon nanotubes, which are "grown" from a process of chemical vapor deposition. The problem is, as the name suggests, carbon nanotubes are small, and the longest grown have been about 14 centimeters (5.5 inches) — that's a big difference to the 100,000-kilometer (62,000-mile) length of a space elevator
Suppose, though, a way is found to manufacture longer nanotubes and mass produce them. Once we have the materials, Stephen Cohen of Vanier College in Quebec, Canada, who is author of "Getting Physics: Nature's Laws as a Guide to Life" and an expert in space elevator design, thinks that the subsequent design phase would take five to 10 years, in parallel with the maturing of the cable material.
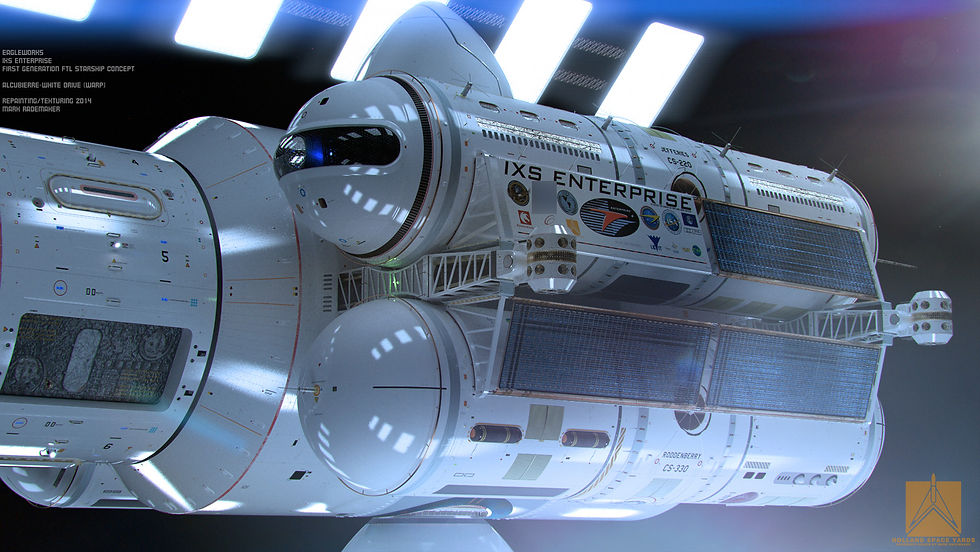
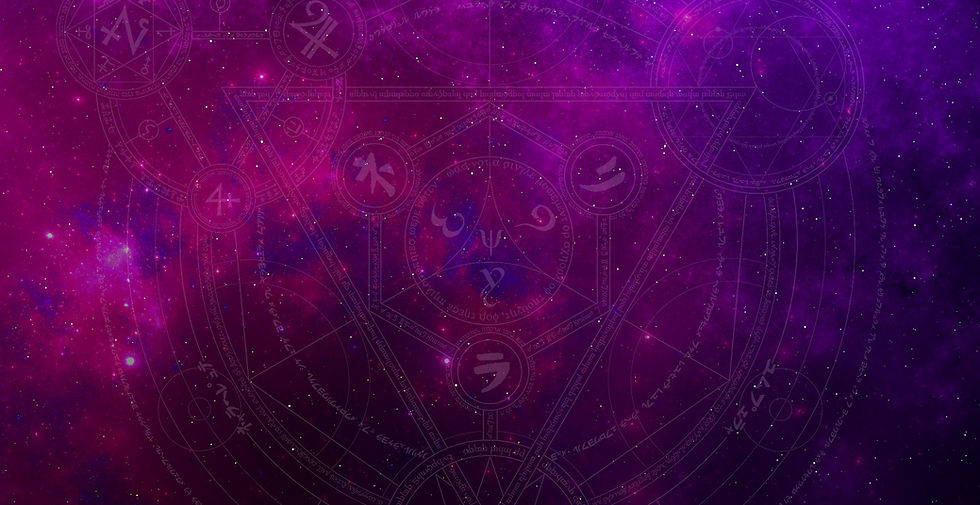
A solar panel in space, above the absorbing effects of the atmosphere, receives about a kilowatt of energy per square meter (10 square feet) of the sun. Solar panels are not 100% efficient, however; currently available commercial models have an efficiency of just 30%, meaning a solar panel in space can realistically produce just 300 watts per square meter. To scale this up to producing 1 gigawatt of energy, which would be the equivalent of a nuclear power station on Earth, would require an enormous array of solar panels, multiple kilometers in diameter, with a mass of 10,000 metric tons. Compare this to the International Space Station, which has a mass of 419 metric tons, and that shows just what a daunting engineering task this would be.
Supposing a solar farm could be built in space, it would be placed in geosynchronous orbit, 35,786 km (22,236 miles) above the Earth. The challenge would then be to keep the microwave beam narrow and on target — you wouldn't want the microwave beam to stray and fry something accidentally. Although lasers instead of microwaves would be easier to direct, laser energy can be absorbed by water vapor in the atmosphere or blocked by clouds, whereas microwaves pass freely through them.
Lasers, though, might be more suitable for space-to-space power transfer. This could extend the life of satellites, for example, but they would have to be built with some kind of receiver to accept the incoming laser power beam. We could also imagine a network of solar farms and relay satellites around the moon, beaming power via lasers to a lunar base on the surface.
Second half of the 21st century: Space elevators
This is an old science fiction concept, first conceived by Russian scientist Konstantin Tsiolkovsky — rather than blasting off in a rocket atop a dangerous column of flame, why not ride into space on an elevator car?
The basic design of a space elevator sounds simple. A thick cable extends from a location within 10 degrees of the Earth's equator up into space. The forces acting on the cable would be fierce, with Earth's gravity trying to pull it down, and the centrifugal force on a mass at the end of the cable in Earth orbit pulling it the other way, keeping it taut. The stresses and tension of this cable would be so great that it would need to be made from a material 50 times stronger than steel, however. The only material strong enough are carbon nanotubes, which are "grown" from a process of chemical vapor deposition. The problem is, as the name suggests, carbon nanotubes are small, and the longest grown have been about 14 centimeters (5.5 inches) — that's a big difference to the 100,000-kilometer (62,000-mile) length of a space elevator
Suppose, though, a way is found to manufacture longer nanotubes and mass produce them. Once we have the materials, Stephen Cohen of Vanier College in Quebec, Canada, who is author of "Getting Physics: Nature's Laws as a Guide to Life" and an expert in space elevator design, thinks that the subsequent design phase would take five to 10 years, in parallel with the maturing of the cable material.
An artist's illustration of a space elevator hub station in space as a transport car rides up the line toward the orbital platform. Solar panels nearby provide power. (Image credit: Obayashi Corp.)
"The cable would be spooled down from geo orbit and eventually fastened to Earth port," Cohen told Space.com. "The ship that unspools it would use fuel to gain altitude and become the far-end anchor some 100,000 kilometers away. Alternatively, a separate ship transports the cable portion above geosynchronous orbit while the original vessel assumes the role of a geo port, which is the true ground central for long term operations. This deployment process could easily take a year."
While we're waiting on the carbon nanotubes, Zephyr Penotre of the University of Cambridge in the U.K. and Emily Sandford of Columbia University in New York think we could be setting about building a space elevator from the moon with current technology. Because of the moon's slower spin rate and lower gravity, they reason that the tension felt by a lunar elevator would not be as great as an elevator coming up from Earth and that carbon polymers such as Zylon would do the trick.
Penoyre and Sandford call their concept a "space-line." Whereas an elevator from Earth would be quite chunky, perhaps ferrying multiple elevator cars up and down simultaneously, the space-line would be a thin wire with a total mass of 40 metric tons. Anchored on the lunar surface, it would dangle toward Earth, stopping somewhere around geosynchronous orbit. So, it wouldn't actually touch Earth or subject it to the destructive tension that doing so would incur.
The idea is that a mission to the moon would launch to geosynchronous orbit, rendezvous with the space-line and ride it the rest of the way to the moon, or to a base at the Earthmoon Lagrange point where the gravity of the Earth and the moon balance out. It would cut the amount of fuel needed to get to the moon by a third; a mission would still have to launch out of Earth gravity well, but the space-line would be solar powered.
Penoyre and Sandford estimate that a prototype would cost billions of dollars, but in the long run it would save money if we are to permanently settle on the moon.
Cohen is looking even further afield, to Mars. "I think a first Mars venture with humans precedes space elevator construction but I would argue that no sustainable colonization of Mars is achievable without an infrastructure like the space elevator," he said. "And so, design and construction phases of the space elevator should probably coincide with the first humans on Mars."
Currently, there are no concrete plans for humans to travel to Mars. Much might depend upon how NASA's Artemis lunar program develops. If a lunar base can be built and crewed by the 2030s, astronauts can learn the skills they'll need to survive on the Red Planet, but first we need to learn how to live on the moon. And to do that, we'll need power, as we'll next discover.
2030s: Nuclear power on the Moon
The moon is tidally locked to the Earth, which means that we always see its same face, the familiar "Man in the Moon." Don't be fooled into thinking that the moon doesn't rotate, however — it does, at the same rate that it revolves around our Earth, which is how it always keeps the same face directed towards us. It takes a little over 27 days — about four weeks — to rotate once, which means (almost) everywhere on the moon sees two weeks of daylight and two weeks of night. The exception is some areas very near the poles, which can see 80% daylight. However, if a lunar base were to be solar powered, it would need 100% daylight, so an alternative power source is needed.
Nations and space agencies around the world are now racing to develop the first nuclear fission reactors for the moon. In February, NASA and the U.S. Department of Energy selected three design proposals for a fission reactor to fly on a future Artemis mission. The reactor has the design specification of producing 40 kilowatts for at least 10 years on the lunar surface.
Meanwhile, the Russians and the Chinese have announced that they are jointly planning an International Lunar Research Station with a nuclear reactor, to launch between 2033 and 2035. However, they admit they have not yet come up with a way of cooling the reactor.
"The major issue is what to do with all that waste heat since there is no air on the Moon to convect it away," Simon Middleburgh of the Nuclear Futures Institute at Bangor University in Wales, told Space.com.
Middleburgh is working with the U.K. Space Agency and Rolls-Royce to develop a nuclear fission reactor that could fly to the moon on a future mission. Rolls-Royce have considerable experience working with nuclear reactors, since they outfit the U.K.'s nuclear submarines with them.
"The aim for the reactor energy output would be of the order of 100–300 kilowatts in combined heat and electrical power – both of which would be extremely useful up there [on the moon],” Middleburgh said. "This is an enormous amount of power compared to previous missions, and as the site [for a lunar base] grows, we may want to build a second or third system that will also provide assurance of energy supply. But we won't be building 100 megawatt systems any time soon."
2070s-2120s: Interstellar travel
It took nine years for the fastest ever space mission, NASA's New Horizons, to reach Pluto at a distance of 34 astronomical units (AU) from the sun. For context, an astronomical unit is the average distance between Earth and the sun. The closest star to the sun is Proxima Centauri. It's 268,779 AU (4.2 light-years) from the Sun. Having passed Pluto, New Horizons reached a velocity of 84,000 km per hour (52,000 mph) and it would take about 80,000 years to reach the distance of Proxima Centauri.
Clearly, we're going to need to find a faster way if we ever hope to travel between the stars, but there are people working on the problem. In the 1970s, members of the British Interplanetary Society developed Project Daedalus, which was plans for a two-stage nuclear-fusion powered but uncrewed starship that could ultimately reach 12% of the speed of light.
In 2016, the Breakthrough Foundation initiated Project Starshot, the concept of which is to drive a fleet of tiny spacecraft, called StarChips, attached to light sails that are pushed up to 20% of the speed of light by powerful laser. They would reach Proxima within decades rather than centuries. Just one problem though: the laser energy output must be up to 100 gigawatts — the equivalent of 100 nuclear power stations.
Related Stories:
— Roller coaster tech could help NASA’s Artemis moon astronauts in case of a launch emergency
— Earth is a spaceship like the ISS — let me explain
— How satellite data has proven climate change is a climate crisis
To solve this problem, we could combine futuristic technologies — the solar farms that could be built in orbit to harness solar energy could instead be used to power the lasers. It's all technology we have now, but on a scale far in advance of what we currently have. The stars are there waiting for us — but it seems they might have to wait a little while longer.
Right now, all we have is Earth.

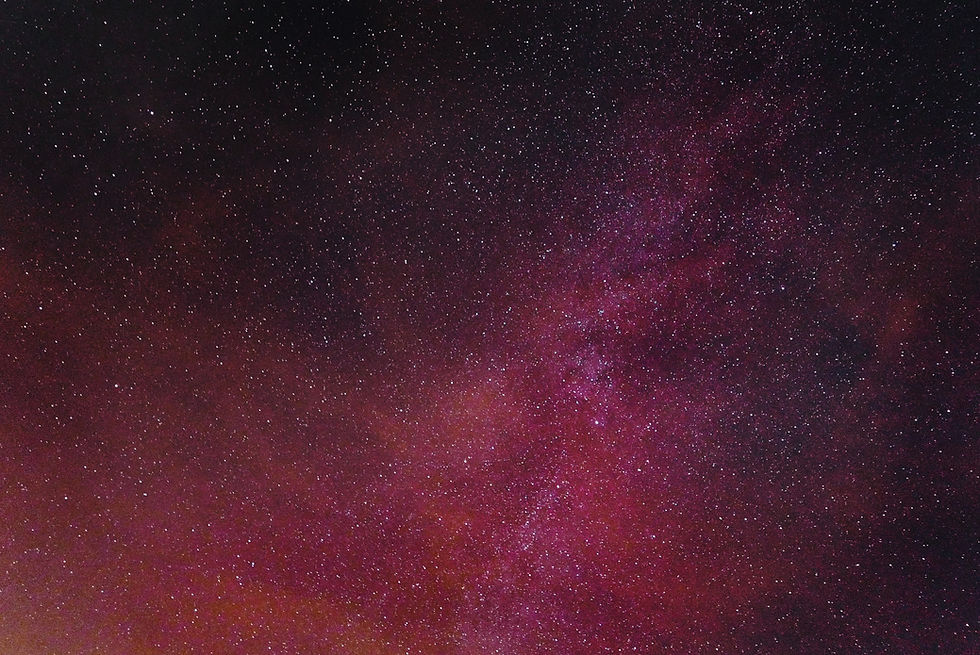
Robert Zubrin, Presiden of the Mars Society, has developed an updated Mars plan that builds on his original Mars Direct proposal. This new plan integrates modern spaceflight technologies, such as SpaceX’s Starship, with his core principles of cost-effectiveness, efficiency, and the use of local Martian resources. Central to this vision is a conceptual spacecraft referred to as the “Starboat,” which likely represents a smaller, specialized vehicle designed to enhance the feasibility of human missions to Mars.
Overview of the Plan
Zubrin’s new Mars plan aims to enable human exploration and eventual colonization of Mars within the next decade. It emphasizes:
Cost-effectiveness: Reducing the financial burden of Mars missions by optimizing spacecraft design and mission architecture.
In-situ Resource Utilization (ISRU): Using Mars’ own resources—such as atmospheric carbon dioxide—to produce fuel and supplies, minimizing the need for large payloads launched from Earth.
Sustainability: Establishing infrastructure on Mars to support a permanent human presence over time.
The plan leverages the capabilities of SpaceX’s Starship, a powerful and reusable launch vehicle, while addressing some of its practical challenges for Mars missions. Zubrin recommends making a mini-Starship called Starboat.
The Starboat – Mini Starship
Starboat likely refers to a smaller, more efficient spacecraft that Zubrin envisions as part of his updated mission architecture.
Starboat would be a vehicle of similar type to the current SpaceX Starship but scaled down by about a factor of five in mass.
It could do a direct return from the Mars surface to Earth using 120 tons of propellant or perform a low-Mars-orbit rendezvous using just 50 tons of propellant, with a single tanker in low Mars orbit being able to support five such return flights. It could also be lifted to Earth orbit fully fueled by a single Starship and sent directly to Mars with five tons of cargo without any Earth-orbit refueling, or 25 tons of cargo with a single tanker refueling.
Here’s how it fits into the plan:
Integration with Starship:
Heavy Lift to Low Earth Orbit (LEO): The Starship serves as the initial launch vehicle, carrying payloads—including the “Starboat”—from Earth to LEO. Its reusability and high payload capacity make it ideal for this role.
Staging for Mars: Once in LEO, the Starboat would be deployed for the interplanetary journey to Mars. Unlike the Starship, which is designed for direct Earth-to-Mars flights in SpaceX’s plans, the “Starboat” is a smaller vehicle optimized for efficiency.
Mars Landing and Operations
Efficient Design: The Starboat needs five times less propellant than the Starship for landing on Mars and returning to orbit. For comparison, refueling the Starship on Mars requires approximately 600 tons of propellant, while Zubrin’s Mars Direct plan uses vehicles needing only about 120 tons.
By a rough estimate, to make the 600 metric tons of propellant required to refuel the Starship once on Mars within a year and a half would require a power source with an average round-the-clock output of 600 kilowatts. A solar array that could do that would cover 60,000 square meters — that’s over 13 football fields in size — and weigh about 240 metric tons. It would require three Starship flights just to deliver such a solar array to Mars, and it would then be a major burden to deploy and maintain. A more practical alternative would be to use nuclear power. We could imagine a plausible reactor design at this power level with a mass of about ten tons.
Alternatively, instead of putting a tanker in low Mars orbit, a Starship fully fitted out for crew could be stationed there, and the Starboat only employed as a reusable shuttle between the surface and orbit. In that case, the plan could retain the ability to employ twenty-person crews, as they could ride out and land Mars along with 100 tons of freight on a standard Starship, only needing to accept the closer quarters on the smaller vehicle during a short Mars-to-orbit flight on the return leg.
The development of the Starboat would also fix the excessive launch problem with the SpaceX Artemis mission plan. The current plan requires 200 tons of propellant to be delivered to low lunar orbit to fuel the Starship on a roundtrip sortie to the lunar surface. At one fifth the size, a Starboat could make the same trip with only 40 tons of fuel. Similarly, the propellant requirement for a round trip from the Gateway to the lunar surface would be reduced from 400 tons to 80. And this could be further reduced by another factor of four when and if lunar oxygen production becomes operational.
The Starboat could also serve as the upper stage of a reusable first-stage booster in the same class as the Falcon-9, Neutron, and New Glenn boosters, thereby creating a fully reusable medium lift system capable of performing many important supporting mission roles.
The Starboat gives Mars base crew with global mobility. Mars is a planet with a surface area equal to all the continents of the Earth put together. It cannot be explored from a single base using slow moving ground vehicles with limited range. To explore Mars competently, we need worldwide access and the ability to travel rapidly across distances of continental scale. With 50, or better yet, 100 tons of propellant, the Starboat could give us this capability in spades.
If additional Starships were landed to establish refueling bases scattered at long distances across the planet, more such explorable regions could be opened up. Nine such refueling stations would provide coverage of the entire world.
ISRU Compatibility: The Starboat would utilize ISRU to produce methane and oxygen from Martian resources, enabling it to refuel on the surface for the return trip. This aligns with Zubrin’s philosophy of minimizing Earth-launched mass.
Mission Architecture:
Modular Approach: The plan may involve multiple Starboats serving different roles, such as a lander for crew and cargo or an Earth Return Vehicle (ERV) for the journey back. This mirrors the modular design of Mars Direct, which separates habitat and return vehicles.
Scalability: Each mission could leave behind equipment and habitats, gradually building a sustainable base on Mars.
Addressing Challenges
Zubrin has critiqued SpaceX’s original Starship plan, particularly the challenge of producing vast amounts of propellant on Mars. The Starboat addresses this by:
Reducing the scale of vehicles involved in Mars landings and returns, making refueling more manageable.
Allowing the Starship to focus on what it does best—delivering heavy payloads to LEO—while the Starboat handles the interplanetary and surface phases.
Starboat could also act as reusable upper stage for Falcon 9, Falcon Heavy, Neutron and Blue Origin New Glenn.
Starboat’s Martian mobility
Starboat can reach low Mars orbit using 50 tons of propellant. That means that using 50 tons or less, it could launch from anywhere on Mars to land anywhere else in less than an hour. That’s one way. But it could also go out and back 1,000 km using 50 tons of propellant, or 2,200 km using 100 tons. Thus, employing a Starboat as a sortie vehicle, a single base could support the exploration of a region 4,400 km in diameter, enclosing some 16 million square kilometers of land.
Using Starboat as an orbit-to-surface ferry for both Mars and the Moon
Traveling from the Martian surface to Mars orbit requires a rocket-propelled velocity change (known as delta-V) of 3.8 km/s. However, it would only need a delta-V of about 0.3 km/s to come down and land — because it can slow down using Mars’s atmosphere — for a total roundtrip delta-V of 4.1 km/s. This is nearly the same as the 3.8 km/s delta-V needed to travel roundtrip from low lunar orbit to the lunar surface.
Thus a Starboat designed for use as an orbit-to-surface ferry on Mars could readily serve the same function on the Moon. It is true that mission performance could be potentially optimized further by creating specialized Starboat designs for each of its potential roles. For example, a Starboat used only for lunar landing and ascent could be built lighter as it would not need thermal protection, while the range of one used for surface-to-surface travel on Mars could be extended by adding wings. But creating more types of specialized flight vehicles would add to the program’s development cost. The question then becomes where across a spectrum ranging from generalization to specialization the program should place its bets.
Elon Musk has argued for a one-size fits all Starship-only mission architecture. This offers maximum simplicity but imposes great costs. By going to a two-size fits all Starship-and-Starboat plan to implement both Mars and lunar missions, 80 percent of these costs can be eliminated.
